Translate this page into:
Regenerative medicine in aesthetics
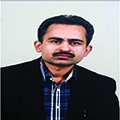
-
Received: ,
Accepted: ,
How to cite this article: Mehta H, Bishnoi A, Dogra S. Regenerative medicine in aesthetics. CosmoDerma 2022;2:41.
Abstract
Regenerative medicine refers to the restoration of the form and function of damaged and diseased tissues by upregulation of natural regenerative processes present in the human body. Applications of regenerative medicine in dermatology are numerous, ranging from the acceleration of wound healing, hair restoration, mesenchymal stem cell augmented fat transfer, skin rejuvenation, enhancing results, and reducing downtime postprocedure and postlaser, etc. In modern aesthetic practice, the most prominent among current regenerative treatments are platelet-rich plasma (PRP), stem cells, growth factors, and most recently, exosomes. Most of the modalities available at present lack high-quality evidence supporting their use and good quality clinical trials are required for the optimization of cellular source, dose, and administration intervals before these modalities are deemed acceptable for use at a wider scale.
Keywords
Aesthetics
Regenerative
Stem cells
Platelet-rich plasma
Exosomes
REGENERATIVE MEDICINE IN AESTHETICS
Regenerative medicine can be defined as “an interdisciplinary field of research and clinical applications focused on the repair, replacement or regeneration of cells, tissues or organs to restore impaired function resulting from any cause, including congenital defects, disease, trauma, and aging.” The idea behind regenerative medicine is to restore form and function to damaged and diseased tissues through biological approaches. Modern regenerative treatments work at the cellular level to upregulate natural regenerative processes present in the human body. Applications of regenerative medicine in dermatology are numerous, ranging from the acceleration of wound healing with platelet-rich fibrin (PRF), hair restoration, skin rejuvenation, mesenchymal stem cell augmented fat transfer, enhancing results, and reducing downtime postprocedure and postlaser, etc.
Regenerative medicine aims at understanding the cell to cell interactions and what drives them, in order to harness them for therapeutic use without causing injury. Procedures such as radiofrequency ablation and carboxytherapy employ natural healing mechanisms of the skin and may be considered a form of regenerative therapy. In modern aesthetic practice, the most prominent among current regenerative treatments are platelet-rich plasma (PRP), stem cells, growth factors, and most recently, exosomes. The idea is to develop safe and effective therapies which produce desired results either alone or in combination with existing therapies. Regenerative medicine is among the most rapidly growing branches of science, and knowledge regarding the basic principles of regenerative aesthetics is imperative among dermatologists and aesthetic practitioners.
STEM CELLS
The cardinal properties of stem cells, that is, self-renewal and plasticity have been utilized in the field of regenerative medicine. According to their differentiation potential, they may be categorized into totipotent, pluripotent, multipotent, and unipotent stem cells.[1] Totipotent and pluripotent stem cells are only found in the embryo and can differentiate into cell lines of all three germ layers. Derivation of embryonic stem cells (ESCs) requires disruption of the early embryo and is hence associated with several ethical concerns. Somatic cells from adults may be reprogrammed by activation of certain transcription factors to generate induced pluripotent stem cells.[2] Adult stem cells include multipotent and unipotent stem cells which have gained popularity in recent times due to their role in tissue maintenance and injury repair.
Umbilical cord blood is a source of multipotent stem cells that does not require surgical intervention for retrieval as it is obtained after clamping of the umbilical cord.[3] Sources of autologous stem cells include adipose-derived stem cells (ADSCs), hair follicle stem cells (HFSCs), dermal fibroblast-derived stem cells, and melanocyte stem cells.[4] ADSCs represent the most popular stem cells utilized in aesthetics due to their abundance, safety, and ease of harvesting.[5] A stromal vascular fraction (SVF) is obtained by centrifugation of aspirate obtained via liposuction.[6] In addition to adipocyte stem cells, SVF consists of preadipocytes, pericytes, hematopoietic progenitor cells, fibroblasts, myocytes, monocytes, endothelial cells, macrophages, and lymphocytes. ADSCs may be isolated from SVF or the SVF may be used directly for aesthetic procedures.
Stem cells in hair restoration
Stem cells are present in hair follicle bulge and dermal papilla and are essential for the normal hair cycle. Exogenous stem cells are an exciting approach to the management of androgenetic alopecia (AGA) due to their potential role in hair growth and regeneration. Several studies have utilized ADSCs as a remedy for AGA. A pilot case series of nine patients utilizing SVF-enhanced autologous fat transfer reported a 23% increase in hair density after 24 weeks.[7] A large study of 71 AGA patients (STYLE trial) compared two doses of ADSCs with autologous fat transfer and saline.[8] Low dose ADSCs were found to significantly improve hair density in the subgroup with early hair loss in this study. Topically applied ADSCs have also shown promise as a therapy for AGA.[9]
Other studies have utilized an ADSC-conditioned medium, which comprises a lyophilized form of protein secreted from cultured ADSCs amassed from healthy adults. This medium is rich in growth factors that enhance the longevity and multiplication of dermal papilla cells.[10] A significant increase in hair density on trichogram was noted after 3 monthly injections of this protein extract and noticeable improvements were seen clinically after 4-5 months.[11]
Other stem cell sources that have been used for AGA include HFSCs and bone marrow-derived mononuclear cells. HFSCs have been obtained by splitting and centrifugation of scalp biopsies, and a significant increase in hair count and density was observed as compared to placebo in split-scalp studies after three injections administered 45 days apart.[12] No significant difference in stem cell count and hair density was noted in a study that compared autologous HFSCs and bone marrow-derived mononuclear cells as stem cell sources.[13]
Stem cells in skin rejuvenation
ADSCs have been demonstrated to have a whitening effect, anti-oxidant action, and stimulate fibroblast proliferation in preclinical studies, suggesting their possible role in the treatment of skin aging.[14-17] This has been translated to clinical improvement in several studies, most of which combined stem cells with microneedling.[18] Zhou et al. compared the combination of ADSCs and fractional carbon dioxide laser with fractional carbon dioxide laser alone in a split-face trial of 13 patients with acne scars and nine patients with skin rejuvenation needs.[19] A higher improvement in patient satisfaction, skin elasticity, and hydration was noted in the combination group. Recently, nano fat emulsions have been utilized for facial rejuvenation.[20] These are obtained by mechanical emulsification of liposuction aspirate to yield a liquid solution. Other stem cells that have been utilized for facial rejuvenation include ESCs and amniotic membrane stem cells.[21,22]
Stem cells in wound healing
Stem cells possess the ability to induce cellular differentiation, regeneration, ability to release of growth factors, and immunomodulation. These properties may be useful in the management of chronic nonhealing ulcers. Among various cutaneous stem cell sources, SVF from adipose tissue has been used widely, due to its ability to differentiate into different components that may expedite wound healing, such as hematopoietic stem cells, fibroblasts, pericytes, endothelial cells, and preadipocytes. Garg et al. described the successful use of a combination of erbium YAG laser, PRP, and autologous fat transplantation in a patient with a recurrent trophic ulcer.[23]
Other dermatologic disorders in which stem cell therapy has been utilized include pemphigus, systemic sclerosis, systemic lupus erythematosus, psoriasis, vitiligo, and epidermolysis bullosa.[24] It must be noted that stem cell therapies are still in their nascent stages. Potential adverse effects such as rejection, malignant potential, uncontrolled proliferation, cross-contamination with other cell lineages, and hyperimmune response cannot be ignored. No standard protocols are available for obtaining autologous stem cells, and as a result standardization and quality remain an issue. Large-scale studies and randomized controlled trials are necessary, along with strict regulatory oversight. Stem cells are currently not approved for commercial use in India and their use outside the purview of clinical trials is prohibited. Among dermatological disorders, the use of Hematopoetic Stem Cell Transplantation (HSCT) is permitted for children (but not adults) with systemic sclerosis.[24]
GROWTH FACTORS
Growth factors are chemical messengers that act by modifying the cellular microenvironment to enhance cell growth, differentiation, and tissue repair. PRP is a platelet concentrate derived from whole blood. It is rich in growth factors that modulate biological activities, including stimulation of stem cell proliferation and differentiation, angiogenesis, and collagen production. The growth factors secreted from PRP include (but are not limited to) transforming growth factor-β, platelet-derived growth factor, insulin-like growth factor-1 (IGF-1), hepatocyte growth factor (HGF), keratinocyte growth factor (KGF), epidermal growth factor and vascular endothelial growth factor. The biological characteristics of PRP depend upon the concentration of platelets and the method of preparation. Platelet concentration up to five times is generally considered adequate for its biological action, with higher concentration not necessarily producing better results.[25] No standard guidelines have been formulated for the preparation of PRP. On the basis of composition, PRP has been classified into pure PRP, leukocyte and PRP, pure PRF, and leukocyte and PRF.[26] PRP is currently being used in aesthetics for skin rejuvenation, hair restoration, and scar repair, usually combined with other procedures such as microneedling.
Growth factors in hair restoration
AGA is characterized by premature termination of the anagen phase of the hair cycle due to dysregulation of the β-catenin/WNT pathway. Growth factors in PRP are individually known to play important roles in the regulation of the hair cycle.[27-29] They act either by regulating of β-catenin/WNT pathway or by providing a permissive microenvironment for hair growth. The therapeutic efficacy of PRP in patients with AGA has been demonstrated in several clinical trials. A quantitative meta-analysis included 17 trials of PRP therapy in AGA, including both male and female AGA, monotherapy, and combination therapy. A significant increase in hair density was noted, from 141.9 α 108.2 hairs/cm2 at baseline to 177.5 α 129.7 hairs/cm2 at the end of the treatment period (P = 0.0004).[30] A significant positive correlation was observed between the number of treatment sessions per month and hair regrowth (r = 0.5, P = 0.03), while mean age was negatively correlated with the percentage change in hair density (r = -0.56, P = 0.016).[30] Most studies that recruited both men and women indicate that PRP treatment may be more effective in male AGA. Several randomized controlled trials have demonstrated synergistic effects of the combination of PRP with oral finasteride and topical minoxidil.[31-34] Overall, the evidence suggests that PRP is a safe and effective treatment for hair loss due to AGA.
Growth factors in skin rejuvenation
Tissue regeneration properties of PRP have been utilized for facial rejuvenation, both topically and as intradermal injections. Most of the clinical benefits have been reported by uncontrolled studies such as case reports and case series, many of which have failed to describe the method of PRP preparation and activation and lacked clearly defined endpoints. Efficacy in controlled studies with a high level of evidence has been less promising. A recent split-face study of 19 women compared the efficacy of lyophilized PRP with saline injection.[35] A higher increase in dermal thickness was observed with saline solution, with no difference in collagen synthesis among the two arms. The synergistic effect of PRP with hyaluronic acid was reported by another large trial, and significant improvement in skin elasticity and facial appearance was noted with the combination as compared to either therapy alone.[36] Increased concentration of growth factors has been observed to improve efficacy, although not in direct proportion to the concentration.[37] Microneedling with PRP and PRP injection can be combined, as the former improves skin texture while the latter improves volume by stimulating collagen production.
Growth factors in acne scars
Several studies have utilized PRP as an adjunctive treatment to microneedling and fractional carbon dioxide laser in acne scarring. A systematic review analyzing the utility of PRP in acne scarring concluded that two to three consecutive monthly sessions of PRP in combination with fractional carbon dioxide laser may improve the results and reduce postlaser downtime.[38] The evidence in support of microneedling and PRP combination is however scarce. Although some studies have reported improved results with a combination,[39-41] others have shown no added benefits.[42,43] It is notable that patients subjectively report higher satisfaction rates with a combination of PRP and microneedling owing to the reduction in post-procedure erythema and edema. Objective evidence regarding improvement of acne scarring with the combination is lacking.
Considered one of the safest aesthetic procedures and cures for all, the future of PRP is quite promising. The major limitation in the evaluation of therapeutic efficacy of PRP is the lack of standardized protocol for PRP preparation and activation. Little is known about the biological action and tissue interactions of PRP. The need for repeated injections and lack of long-term safety and efficacy data are other limitations to PRP therapy.
EXOSOMES
Exosomes are cell-originated vesicular structures with a lipid bilayer membrane containing proteins, nucleic acids, and lipids secreted by various cells. They have been demonstrated to play an important role in cell-to-cell communication and modulation of cellular replication, differentiation, and apoptosis. Endogenic exosomes in the skin have been implicated in various chronic inflammatory skin diseases.[44] Exosomes derived from stem cells may have a potential therapeutic role in hair restoration by regulation of hair cycle, in skin pigmentation by modulation of melanin synthesis, in skin rejuvenation and scar repair by alteration of collagen synthesis.
Exosomes in scar repair
Exosome-mediated intercellular communication among macrophages, fibrocytes, and fibroblasts/myofibroblasts are essential for scar formation.[45,46] Blockade of exosome generation by M2 macrophages has been successfully used to suppress hypertrophic scar formation.[45] Hu et al. demonstrated the wound healing potential of adipose mesenchymal stem cells (ASC) derived exosomes in a mouse model.[47] ASCs-derived exosomes were internalized by fibroblasts and optimized their migration and replicative capabilities along with collagen and elastin production. Further exploration of the role of ASCs-derived exosomes revealed their role in extracellular matrix remodeling and scarless wound repair, by regulation of collagen type III to type I ratio, transforming growth factor (TGF)—β3 to TGF-β1 ratio, and matrix metalloproteinase-3 to tissue inhibitor of metalloproteinase-1 ratio, and by modulation of fibroblast differentiation.[48] Exosomes derived from other types of mesenchymal stem cells (MSCs), such as induced pleuripotent stem cells,[49] human menstrual blood-MSCs,[50] human umbilical cord MSCs,[51] human umbilical cord plasma cells[52] have also been demonstrated to modulate scar repair in mouse models.
Exosomes in hair restoration
Hair follicle stem cells and dermal papilla cells (DPCs) are essential for hair growth and maintenance of the normal hair cycle. Exosomes derived from DPCs have been observed to accelerate the onset of the anagen phase and delay catagen in mouse model.[53] Stimulation of β-catenin and the sonic hedgehog was observed, with enhanced stimulation and migration of outer root sheath cells. Increased hair shaft elongation was noted in another study, with increased expression of growth factors such as HGF, IGF-1, and KGF in DPCs.[54] DPC-derived exosomes may be a promising agent for the prevention and treatment of hair loss. Suspension mediums such as cryogel wound dressings[55] and hyaluronic acid patches [56] have been utilized to increase the efficiency and prolong the action of exosome injections in promoting hair regrowth.
Exosomes in skin rejuvenation
Regulation of the properties of human dermal fibroblasts (HDF) via exosomes may potentially have a role as an anti-aging therapy. Exosomes derived from HDF spheroids led to increased production of procollagen type I and decreased expression of matrix metalloproteinase-1 in a nude mice photoaging model, via upregulation of TGF-β and down-regulation of tumor necrosis factor-α.[57] Human-induced pleuripotent cell-derived exosomes amended changes of photoaging in HDFs by increasing collagen I production, reduction of expression of MMP-1/3, and downregulation of senescence-associated β-galactosidase production.[58] Embryonic stem cell-derived exosomes have exhibited amelioration of senescence via modulation of the TGF-β receptor 2 pathway in HDFs.[59]
Exosomes in skin pigmentation
Exosomes derived from keratinocytes have an important role in pigment production by melanocytes.[60] Keratinocyte derived exosomes had a high concentration of miR-330-5p which led to an increased expression of miR-330-5p in melanocytes, with the end result of decreased melanin production and tyrosinase gene expression.[60] Phototype-dependence and UV-B mediated modulation of miRNA profile of keratinocyte-derived exosomes was reported by Cicero et al.[61] Kim et al. reported stimulation of melanin production by inhibition of MITF expression by miR-675 from keratinocyte-derived exosomes.[62] Further studies on exosome-mediated interaction between keratinocytes and melanocytes present an exciting prospect for the regulation of pigment production in healthy and diseased states.
The promising field of exosomes is however not devoid of limitations. Lack of standardization in isolation, production, yield, and identification compromises the qualitative analysis of exosomes. Complete isolation of exosomes from the source is difficult, improvement in isolation techniques is desirable to improve yield and purity. The existing literature only pertains to preclinical studies and hence may not be predictive of human skin. The mechanism of exosomes and the biological information transmitted by them is quite complex and not entirely understood at present. As demonstrated by the pre-clinical data, exosomes derived from different sources may have similar actions, which type of exosome produces higher clinical efficacy with fewer side effects remains to be explored.
CONCLUSION
The rapidly expanding realm of regenerative medicine is undoubtedly going to play an important role in the field of aesthetics. There is an increasing demand from patients as well as physicians for effective aesthetic procedures that are safe and harness the natural regenerative mechanisms of the human body. Most of the modalities available at present lack high-quality evidence supporting their use and good quality clinical trials are required for the optimization of cellular source, dose, and administration intervals before these modalities are deemed acceptable for use at a wider scale. We offer key insights into this exciting branch of science that may be the future of aesthetics in the coming decades.
Declaration of patient consent
Patient consent not required as there are no patients in this study.
Financial support and sponsorship
Nil.
Conflict of interest
Author Sunil Dogra is the Deputy Editor of the journal.
References
- Introduction to stem cells and regenerative medicine. Respiration. 2013;85:3-10.
- [CrossRef] [PubMed] [Google Scholar]
- Induction of pluripotent stem cells from mouse embryonic and adult fibroblast cultures by defined factors. Cell. 2006;126:663-76.
- [CrossRef] [PubMed] [Google Scholar]
- Human umbilical cord blood as a potential source of transplantable hematopoietic stem/progenitor cells. Proc Natl Acad Sci U S A. 1989;86:3828-32.
- [CrossRef] [PubMed] [Google Scholar]
- Adipose-derived cellular and cell-derived regenerative therapies in dermatology and aesthetic rejuvenation. Ageing Res Rev. 2019;54:100933.
- [CrossRef] [PubMed] [Google Scholar]
- Multilineage cells from human adipose tissue: Implications for cell-based therapies. Tissue Eng. 2001;7:211-28.
- [CrossRef] [PubMed] [Google Scholar]
- Hair follicle growth by stromal vascular fraction-enhanced adipose transplantation in baldness. Stem Cells Cloning. 2017;10:1-10.
- [CrossRef] [PubMed] [Google Scholar]
- Cell enriched autologous fat grafts to follicular niche improves hair regrowth in early androgenetic alopecia. Aesthet Surg J. 2020;40:Np328-np39.
- [CrossRef] [PubMed] [Google Scholar]
- A randomized, double-blind, vehicle-controlled clinical study of hair regeneration using adipose-derived stem cell constituent extract in androgenetic alopecia. Stem Cells Transl Med. 2020;9:839-49.
- [CrossRef] [PubMed] [Google Scholar]
- Hair growth promoting effects of adipose tissue-derived stem cells. J Dermatol Sci. 2010;57:134-7.
- [CrossRef] [PubMed] [Google Scholar]
- Hair regeneration therapy: Application of adipose-derived stem cells. Curr Stem Cell Res Ther. 2017;12:531-4.
- [CrossRef] [PubMed] [Google Scholar]
- Autologous micrografts from scalp tissue: Trichoscopic and long-term clinical evaluation in male and female androgenetic alopecia. BioMed Research International. 2020;2020:7397162.
- [CrossRef] [Google Scholar]
- Stem cell therapy as a novel therapeutic intervention for resistant cases of alopecia areata and androgenetic alopecia. J Dermatolog Treat. 2018;29:431-40.
- [CrossRef] [PubMed] [Google Scholar]
- Whitening effect of adipose-derived stem cells: A critical role of TGF-beta 1. Biol Pharm Bull. 2008;31:606-10.
- [CrossRef] [PubMed] [Google Scholar]
- Evidence supporting antioxidant action of adipose-derived stem cells: Protection of human dermal fibroblasts from oxidative stress. J Dermatol Sci. 2008;49:133-42.
- [CrossRef] [PubMed] [Google Scholar]
- Determination of adipose-derived stem cell application on photo-aged fibroblasts, based on paracrine function. Cytotherapy. 2011;13:378-84.
- [CrossRef] [PubMed] [Google Scholar]
- Improvement of photoaged skin wrinkles with cultured human fibroblasts and adipose-derived stem cells: A comparative study. J Plast Reconstr Aesthet Surg. 2015;68:372-81.
- [CrossRef] [PubMed] [Google Scholar]
- Efficacy of protein extracts from medium of adipose-derived stem cells via microneedles on Asian skin. J Cosmet Laser Ther. 2018;20:237-44.
- [CrossRef] [PubMed] [Google Scholar]
- The efficacy of conditioned media of adipose-derived stem cells combined with ablative carbon dioxide fractional resurfacing for atrophic acne scars and skin rejuvenation. J Cosmet Laser Ther. 2016;18:138-48.
- [CrossRef] [PubMed] [Google Scholar]
- Nanofat needling: A novel method for uniform delivery of adipose-derived stromal vascular fraction into the skin. Plast Reconstr Surg. 2019;143:1062-5.
- [CrossRef] [PubMed] [Google Scholar]
- The effects of amniotic membrane stem cell-conditioned medium on photoaging. J Dermatolog Treat. 2019;30:478-82.
- [CrossRef] [PubMed] [Google Scholar]
- Efficacy of microneedling plus human stem cell conditioned medium for skin rejuvenation: A randomized, controlled, blinded split-face study. Ann Dermatol. 2014;26:584-91.
- [CrossRef] [PubMed] [Google Scholar]
- Use of stem cells in in-tervention dermatology and trichology: A new hope. J Stem Cell Res Dev Ther 2019:S1004.
- [CrossRef] [Google Scholar]
- Stem cell therapy in dermatology. Indian J Dermatol Venereol Leprol. 2021;87:753-67.
- [CrossRef] [PubMed] [Google Scholar]
- Platelet-rich plasma: Evidence to support its use. J Oral Maxillofac Surg. 2004;62:489-96.
- [CrossRef] [PubMed] [Google Scholar]
- Classification of platelet concentrates (platelet-rich plasma-PRP, platelet-rich fibrin-PRF) for topical and infiltrative use in orthopedic and sports medicine: Current consensus, clinical implications and perspectives. Muscles Ligaments Tendons J. 2014;4:3-9.
- [PubMed] [Google Scholar]
- Suppression of wnt/β-catenin signaling by EGF receptor is required for hair follicle development. Mol Biol Cell. 2018;29:2784-99.
- [CrossRef] [PubMed] [Google Scholar]
- Androgen induction of follicular epithelial cell growth is mediated via insulin-like growth factor-I from dermal papilla cells. Biochem Biophys Res Commun. 1995;212:988-94.
- [CrossRef] [PubMed] [Google Scholar]
- Fibroblast growth factors stimulate hair growth through β-catenin and Shh expression in C57BL/6 mice. Biomed Res Int. 2015;2015:730139.
- [CrossRef] [PubMed] [Google Scholar]
- Effectiveness of platelet-rich plasma therapy in androgenic alopecia-a meta-analysis. J Pers Med. 2022;12:342.
- [CrossRef] [PubMed] [Google Scholar]
- Platelet-rich plasma in combination with 5% minoxidil topical solution and 1 mg oral finasteride for the treatment of androgenetic alopecia: A randomized placebo-controlled, double-blind, half-head study. Dermatol Surg. 2018;44:126-30.
- [CrossRef] [PubMed] [Google Scholar]
- Comparison of efficacy of platelet-rich plasma therapy with or without topical 5% minoxidil in male-type baldness: A randomized, double-blind placebo control trial. Indian J Dermatol Venereol Leprol. 2020;86:150-7.
- [CrossRef] [PubMed] [Google Scholar]
- Comparative evaluation of the clinical efficacy of PRP-therapy, minoxidil, and their combination with immunohistochemical study of the dynamics of cell proliferation in the treatment of men with androgenetic alopecia. Int J Mol Sci. 2020;21:6516.
- [CrossRef] [PubMed] [Google Scholar]
- Evaluation of adding platelet-rich plasma to combined medical therapy in androgenetic alopecia. J Cosmet Dermatol. 2021;20:1427-34.
- [CrossRef] [PubMed] [Google Scholar]
- The effect of lyophilized platelet rich-plasma on skin aging: A non-randomized, controlled, pilot trial. Arch Dermatol Res. 2021;313:863-71.
- [CrossRef] [PubMed] [Google Scholar]
- Synergistic effects of autologous platelet-rich plasma and hyaluronic acid injections on facial skin rejuvenation. Aesthet Surg J. 2021;41:Np854-np65.
- [CrossRef] [PubMed] [Google Scholar]
- Safety and efficacy of growth factor concentrate in the treatment of nasolabial fold correction: Split face pilot study. Indian J Dermatol. 2015;60:520.
- [CrossRef] [PubMed] [Google Scholar]
- Platelet-rich plasma and its utility in the treatment of acne scars: A systematic review. J Am Acad Dermatol. 2019;80:1730-45.
- [CrossRef] [PubMed] [Google Scholar]
- Combined autologous platelet-rich plasma with microneedling verses microneedling with distilled water in the treatment of atrophic acne scars: A concurrent split-face study. Journal of Cosmetic Dermatology. 2016;15:434-43.
- [CrossRef] [PubMed] [Google Scholar]
- Therapeutic effect of microneedling and autologous platelet-rich plasma in the treatment of atrophic scars: A randomized study. Journal of Cosmetic Dermatology. 2017;16:388-99.
- [CrossRef] [PubMed] [Google Scholar]
- Microneedling combined with platelet-rich plasma or trichloroacetic acid peeling for management of acne scarring: A split-face clinical and histologic comparison. Journal of Cosmetic Dermatology. 2018;17:73-83.
- [CrossRef] [Google Scholar]
- Skin microneedling plus platelet-rich plasma versus skin microneedling alone in the treatment of atrophic post acne scars: A split face comparative study. Journal of Dermatological Treatment. 2018;29:281-6.
- [CrossRef] [PubMed] [Google Scholar]
- Split face comparative study of microneedling with PRP versus microneedling with vitamin C in treating atrophic post acne scars. J Cutan Aesthet Surg. 2014;7:209-12.
- [CrossRef] [PubMed] [Google Scholar]
- Exosomes in chronic inflammatory skin diseases and skin tumors. Exp Dermatol. 2019;28:213-8.
- [CrossRef] [PubMed] [Google Scholar]
- Blockade of lncRNA-ASLNCS5088-enriched exosome generation in M2 macrophages by GW4869 dampens the effect of M2 macrophages on orchestrating fibroblast activation. FASEB J. 2019;33:12200-12.
- [CrossRef] [PubMed] [Google Scholar]
- A flowable placental formulation prevents bleomycin-induced dermal fibrosis in aged mice. Int J Mol Sci. 2020;21:4242.
- [CrossRef] [PubMed] [Google Scholar]
- Exosomes derived from human adipose mensenchymal stem cells accelerates cutaneous wound healing via optimizing the characteristics of fibroblasts. Sci Rep. 2016;6:32993.
- [CrossRef] [PubMed] [Google Scholar]
- Exosomes secreted by human adipose mesenchymal stem cells promote scarless cutaneous repair by regulating extracellular matrix remodelling. Sci Rep. 2017;7:13321.
- [CrossRef] [Google Scholar]
- Exosomes released from human induced pluripotent stem cells-derived MSCs facilitate cutaneous wound healing by promoting collagen synthesis and angiogenesis. J Transl Med. 2015;13:49.
- [CrossRef] [PubMed] [Google Scholar]
- Promising effects of exosomes isolated from menstrual blood-derived mesenchymal stem cell on wound-healing process in diabetic mouse model. J Tissue Eng Regen Med. 2019;13:555-68.
- [CrossRef] [PubMed] [Google Scholar]
- Umbilical cord-derived mesenchymal stem cell-derived exosomal microRNAs suppress myofibroblast differentiation by inhibiting the transforming growth factor-β/SMAD2 pathway during wound healing. Stem Cells Transl Med. 2016;5:1425-39.
- [CrossRef] [PubMed] [Google Scholar]
- Exosomes from human umbilical cord blood accelerate cutaneous wound healing through miR-21-3p-mediated promotion of angiogenesis and fibroblast function. Theranostics. 2018;8:169.
- [CrossRef] [PubMed] [Google Scholar]
- Regulation of hair follicle development by exosomes derived from dermal papilla cells. Biochem Biophys Res Commun. 2018;500:325-32.
- [CrossRef] [PubMed] [Google Scholar]
- Exosomes derived from human dermal papilla cells promote hair growth in cultured human hair follicles and augment the hair-inductive capacity of cultured dermal papilla spheres. Exp Dermatol. 2019;28:854-7.
- [CrossRef] [PubMed] [Google Scholar]
- Exosome laden oxygen releasing antioxidant and antibacterial cryogel wound dressing oxoband alleviate diabetic and infectious wound healing. Biomaterials. 2020;249:120020.
- [CrossRef] [PubMed] [Google Scholar]
- A therapeutic microneedle patch made from hair-derived keratin for promoting hair regrowth. Acs Nano. 2019;13:4354-60.
- [CrossRef] [PubMed] [Google Scholar]
- Needle-free injection of exosomes derived from human dermal fibroblast spheroids ameliorates skin photoaging. ACS nano. 2019;13:11273-82.
- [CrossRef] [PubMed] [Google Scholar]
- Exosomes derived from human induced pluripotent stem cells ameliorate the aging of skin fibroblasts. Int J Mol Sci. 2018;19:1715.
- [CrossRef] [PubMed] [Google Scholar]
- Embryonic stem cell-derived mmu-miR-291a-3p inhibits cellular senescence in human dermal fibroblasts through the TGF-β receptor 2 pathway. J Gerontol A Biol Sci Med Sci. 2019;74:1359-67.
- [CrossRef] [PubMed] [Google Scholar]
- Exosomal mirna derived from keratinocytes regulates pigmentation in melanocytes. J Dermatol Sci. 2019;93:159-67.
- [CrossRef] [PubMed] [Google Scholar]
- Exosomes released by keratinocytes modulate melanocyte pigmentation. Nat Commun. 2015;6:7506.
- [CrossRef] [PubMed] [Google Scholar]
- Reduced mir-675 in exosome in H19 RNA-related melanogenesis via mitf as a direct target. Journal of Investigative Dermatology. 2014;134:1075-82.
- [CrossRef] [PubMed] [Google Scholar]